Temperature effect on the porosity of hydroxyapatite scaffolds and its use in tissue engineering
DOI:
https://doi.org/10.37636/recit.v34213221Keywords:
Hydroxyapatite, porous scaffold, salt leaching methodAbstract
The search for a suitable bone replacement is of great importance due to the difficulty to use autologous transplants. Hence, the objective of this work is to compare the temperature effect on the porosity and average pore diameter of hydroxyapatite porous scaffolds fabricated by the salt leaching method. Hydroxyapatite porous scaffolds fabricated by the salt leaching technique were sintered from ~150 to 1000 °C. Synthesized hydroxyapatite was assessed by X-ray diffraction (XRD). Zeta potential at different temperatures was evaluated. Specimens were characterized using scanning electron microscopy (SEM) and Raman analysis. The results showed that significant porosity (57%) and pore size (49 µm) occurred with a thermal treatment above ~ 850 °C for scaffolds that were pre-sintered at 1050 °C.
Downloads
References
K. Yang et al., “β-Tricalcium phosphate/poly (glycerol sebacate) scaffolds with robust mechanical property for bone tissue engineering,” Mater. Sci. Eng. C, vol. 56, pp. 37–47, Nov. 2015. http://dx.doi.org/10.1016/j.msec.2015.05.083. DOI: https://doi.org/10.1016/j.msec.2015.05.083
Z. Sheikh, C. Sima, and M. Glogauer, “Bone Replacement Materials and Techniques Used for Achieving Vertical Alveolar Bone Augmentation,” Materials, vol. 8, no. 6. 2015. http://dx.doi.org/10.3390/ma8062953. DOI: https://doi.org/10.3390/ma8062953
J. Lindhe. Periodontologia Clinica E Implantologia Odontologica. Médica Panamericana; 2009. https://books.google.com.mx/books?id=69zuJ1qspGwC.
L. J. Villarreal-Gómez et al., “Biocompatibility evaluation of electrospun scaffolds of poly (L-Lactide) with pure and grafted hydroxyapatite,” J. Mex. Chem. Soc., vol. 58, no. 4, pp. 435–443, 2014. http://dx.doi.org/10.29356/jmcs.v58i4.53. DOI: https://doi.org/10.29356/jmcs.v58i4.53
G. Hannink and J. J. C. Arts, “Bioresorbability, porosity and mechanical strength of bone substitutes: What is optimal for bone regeneration?” Injury, vol. 42, pp. S22–S25, Dec. 2016. http://dx.doi.org/10.1016/j.injury.2011.06.008. DOI: https://doi.org/10.1016/j.injury.2011.06.008
C. T. I. Reviews, Biomaterials Science, An Introduction to Materials in Medicine. Cram101, 2016. https://books.google.com.mx/books?id=TdrIAQAAQBAJ.
R. Bosco, J. Van Den Beucken, S. Leeuwenburgh, and J. Jansen, “Surface Engineering for Bone Implants: A Trend from Passive to Active Surfaces,” Coatings, vol. 2, no. 3. 2012. http://dx.doi.org/10.3390/coatings2030095. DOI: https://doi.org/10.3390/coatings2030095
L. J. Villarreal-Gómez et al., In vivo biocompatibility of dental scaffolds for tissue regeneration, vol. 976. 2014. http://dx.doi.org/10.4028/www.scientific.net/AMR.976.191. DOI: https://doi.org/10.4028/www.scientific.net/AMR.976.191
K. Kuroda and M. Okido, “Hydroxyapatite Coating of Titanium Implants Using Hydroprocessing and Evaluation of Their Osteoconductivity,” Bioinorg. Chem. Appl., vol. 2012, no. 1, pp. 1–7, 2012, doi: http://dx.doi.org/10.1155/2012/730693. DOI: https://doi.org/10.1155/2012/730693
C. Berger et al., “Ultrathin Epitaxial Graphite: 2D Electron Gas Properties and a Route toward Graphene-based Nanoelectronics,” J. Phys. Chem. B, vol. 108, no. 52, pp. 19912–19916, Dec. 2004. http://dx.doi.org/10.1021/jp040650f. DOI: https://doi.org/10.1021/jp040650f
L. Chen, Y. Tang, K. Wang, C. Liu, and S. Luo, “Direct electrodeposition of reduced graphene oxide on glassy carbon electrode and its electrochemical application,” Electrochem. commun., vol. 13, no. 2, pp. 133–137, Feb. 2011. http://dx.doi.org/10.1016/j.elecom.2010.11.033. DOI: https://doi.org/10.1016/j.elecom.2010.11.033
J. Shi, X. Li, G. He, L. Zhang, and M. Li, “Electrodeposition of high-capacitance 3D CoS/graphene nanosheets on nickel foam for high-performance aqueous asymmetric supercapacitors,” J. Mater. Chem. A, vol. 3, no. 41, pp. 20619–20626, 2015. http://dx.doi.org/10.1039/C5TA04464B. DOI: https://doi.org/10.1039/C5TA04464B
A. Di Luca et al., “Gradients in pore size enhance the osteogenic differentiation of human mesenchymal stromal cells in three-dimensional scaffolds,” Sci. Rep., vol. 6, p. 22898, Mar. 2016. http://dx.doi.org/10.1038/srep22898. DOI: https://doi.org/10.1038/srep22898
C. M. Murphy and F. J. O’Brien, “Understanding the effect of mean pore size on cell activity in collagen-glycosaminoglycan scaffolds,” Cell Adh. Migr., vol. 4, no. 3, pp. 377–381, Dec. 2010. http://dx.doi.org/10.4161/cam.4.3.11747. DOI: https://doi.org/10.4161/cam.4.3.11747
P. Kasten, I. Beyen, P. Niemeyer, R. Luginbühl, M. Bohner, and W. Richter, “Porosity and pore size of β-tricalcium phosphate scaffold can influence protein production and osteogenic differentiation of human mesenchymal stem cells: An in vitro and in vivo study,” Acta Biomater., vol. 4, no. 6, pp. 1904–1915, Nov. 2008. http://dx.doi.org/10.1016/j.actbio.2008.05.017. DOI: https://doi.org/10.1016/j.actbio.2008.05.017
L. D. Guillen-Romero et al., “Synthetic hydroxyapatite and its use in bioactive coatings,” J. Appl. Biomater. Funct. Mater., vol. 17, no. 1, 2019. http://dx.doi.org/10.1177/2280800018817463. DOI: https://doi.org/10.1177/2280800018817463
J. Wosek, “Fabrication of Composite Polyurethane/Hydroxyapatite Scaffolds Using Solvent-Casting Salt Leaching Technique,” Advances in Materials Science, vol. 15. p. 14, 2015. http://dx.doi.org/10.1515/adms-2015-0003. DOI: https://doi.org/10.1515/adms-2015-0003
S. Fragoso Angeles, R. Vera-Graziano, G. L. Pérez González, A. L. Iglesias, L. E. Gómez Pineda, and L. J. Villarreal-Gómez, “Síntesis y Caracterización de Hidroxiapatita Sintética para la Preparación de Filmes de PLGA/HAp con Potencial Uso en Aplicaciones Biomédicas,” ReCIBE, vol. 7, no. 2, pp. 93–116, 2018. http://recibe.cucei.udg.mx/ojs/index.php/ReCIBE/article/view/94. DOI: https://doi.org/10.32870/recibe.v7i2.94
S. Koutsopoulos, “Synthesis and characterization of hydroxyapatite crystals: a review study on the analytical methods”. J Biomed Mater Res. Vol. 62, Pp. 600–612, 2002. https://doi.org/10.1002/jbm.10280 DOI: https://doi.org/10.1002/jbm.10280
A.F. Khan, M. Awais, A.S. Khan, “Raman spectroscopy of natural bone and synthetic apatites”. Appl Spectrosc Rev. Vol. 48, Pp. 329–355, 2013. https://doi.org/10.1080/05704928.2012.721107 DOI: https://doi.org/10.1080/05704928.2012.721107
G.R. Sauer, W.B. Zunic, J. R. Durig, “Fourier transform Raman spectroscopy of synthetic and biological calcium phosphates”. Calcif Tissue Int. Vol. 54, Pp. 414, 1994. https://doi.org/10.1007/BF00305529 DOI: https://doi.org/10.1007/BF00305529
A.-J. Wang et al., “Effect of sintering on porosity, phase, and surface morphology of spray dried hydroxyapatite microspheres,” J. Biomed. Mater. Res. Part A, vol. 87A, no. 2, pp. 557–562, 2008, http://dx.doi.org/10.1002/jbm.a.31895. DOI: https://doi.org/10.1002/jbm.a.31895
S. V Dorozhkin, “Calcium orthophosphate deposits: Preparation, properties and biomedical applications,” Mater. Sci. Eng. C, vol. 55, pp. 272–326, Oct. 2015, doi: http://dx.doi.org/10.1016/j.msec.2015.05.033. DOI: https://doi.org/10.1016/j.msec.2015.05.033
C. F. C. Brown, J. Yan, T. T. Y. Han, D. M. Marecak, B. G. Amsden, and L. E. Flynn, “Effect of decellularized adipose tissue particle size and cell density on adipose-derived stem cell proliferation and adipogenic differentiation in composite methacrylated chondroitin sulphate hydrogels,” Biomed. Mater., vol. 10, no. 4, p. 45010, 2015, http://dx.doi.org/10.1088/1748-6041/10/4/045010. DOI: https://doi.org/10.1088/1748-6041/10/4/045010
D. N. Misra, Adsorption on and Surface Chemistry of Hydroxyapatite. Springer US, 2013. https://books.google.com.mx/books?id=uDHvBwAAQBAJ.
M. Morga, Z. Adamczyk, and M. Oćwieja, “Stability of silver nanoparticle monolayers determined by in situ streaming potential measurements,” J. Nanoparticle Res., vol. 15, no. 11, p. 2076, Oct. 2013. http://dx.doi.org/10.1007/s11051-013-2076-5. DOI: https://doi.org/10.1007/s11051-013-2076-5
P. de Vos, B. J. de Haan, J. A. A. M. Kamps, M. M. Faas, and T. Kitano, “Zeta-potentials of alginate-PLL capsules: A predictive measure for biocompatibility?” J. Biomed. Mater. Res. Part A, vol. 80A, no. 4, pp. 813–819, 2007. http://dx.doi.org/10.1002/jbm.a.30979. DOI: https://doi.org/10.1002/jbm.a.30979
D. Malina, K. Biernat, and A. Sobczak-Kupiec, “Studies on sintering process of synthetic hydroxyapatite,” Acta Biochim. Pol., vol. 60, no. 4, pp. 851–855, 2013. http://www.actabp.pl/pdf/4_2013/851.pdf. DOI: https://doi.org/10.18388/abp.2013_2071
J. González Ocampo, D. M. Escobar Sierra, and C. P. Ossa Orozco, “Métodos de fabricación de cuerpos porosos de hidroxiapatita, revisión del estado del arte,” Revista ION, vol. 27. scieloco, pp. 55–70, 2014. https://revistas.uis.edu.co/index.php/revistaion
M. Sadat-Shojai, M.-T. Khorasani, E. Dinpanah-Khoshdargi, and A. Jamshidi, “Synthesis methods for nanosized hydroxyapatite with diverse structures,” Acta Biomater., vol. 9, no. 8, pp. 7591–7621, Aug. 2013. http://dx.doi.org/10.1016/j.actbio.2013.04.012. DOI: https://doi.org/10.1016/j.actbio.2013.04.012
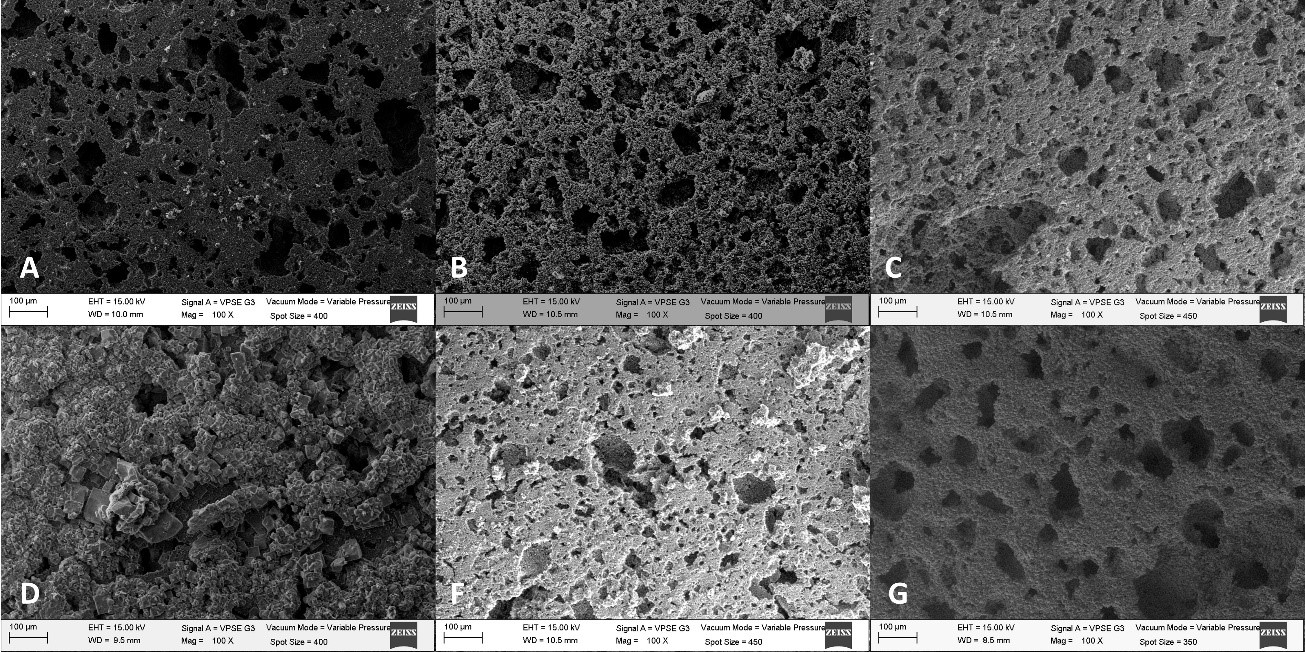
Published
How to Cite
Issue
Section
Categories
License
Copyright (c) 2020 Vareska Lucero Zarate-Córdova, Mercedes Teresita Oropeza-Guzmán, Eduardo Alberto López-Maldonado, Dra. Ana Leticia Iglesias, Theodore Ng, Eduardo Serena-Gómez, Dra. Graciela Lizeth Pérez González, Luis Jesús Villarreal-Gómez

This work is licensed under a Creative Commons Attribution 4.0 International License.
The authors who publish in this journal accept the following conditions:
The authors retain the copyright and assign to the journal the right of the first publication, with the work registered with the Creative Commons Attribution license 4.0, which allows third parties to use what is published as long as they mention the authorship of the work and the first publication in this magazine.
Authors may make other independent and additional contractual agreements for the non-exclusive distribution of the version of the article published in this journal (eg, include it in an institutional repository or publish it in a book) as long as they clearly indicate that the work it was first published in this magazine.
Authors are allowed and encouraged to share their work online (for example: in institutional repositories or personal web pages) before and during the manuscript submission process, as it can lead to productive exchanges, greater and more quick citation of published work (see The Effect of Open Access).