Relationship between growth and temperature at the tip of the fatigue crack in AISI 1018 steel
DOI:
https://doi.org/10.37636/recit.v6n1e245Keywords:
Crack growth, Temperature, Metal fatigue, Thermocouples, Thermographic cameraAbstract
In this work, a study dealing with the correlation between the fatigue crack growth behavior and the temperature evolution at the tip of the fatigue crack of the AISI 1018 steel is presented. Both the crack length and the temperature at the crack tip were experimentally obtained from a fatigue test carried out according to the ASTM E647; the temperature at the crack tip was simultaneously acquired by thermocouples and infrared thermography, while the crack length was acquired through a microscope. The experimental data were processed aimed at plotting the curves of the temperature against cycles as well as the crack length against cycles in order to be able to correlate the information and, therefore, to obtain by linear regression of the experimental data a model to correlate the temperature at the crack tip to the crack length. The results showed that, on one hand, the linear regression is in good agreement with the experimental data, and, on the other hand, the proposed model allows for estimating the trend and the magnitude of the temperature when the crack grows.
Downloads
References
W. Schütz, “A history of fatigue,” Engineering Fracture Mechanics, vol. 54, no. 2, pp. 263–300, 1996. https://doi.org/10.1016/0013-7944(95)00178-6 DOI: https://doi.org/10.1016/0013-7944(95)00178-6
U. Zerbst, M. Madia, C. Klinger, D. Bettge, and Y. Murakami, “Defects as a root cause of fatigue failure of metallic components. I: Basic aspects,” Engineering Failure Analysis, vol. 97, pp. 777–792, 2019. http://dx.doi.org/10.1016/j.engfailanal.2019.01.055 DOI: https://doi.org/10.1016/j.engfailanal.2019.01.055
C. S. Pande, “Fundamentals of Fatigue Crack Initiation and propagation: Some thoughts,” Fatigue of Materials II, pp. 3–15, 2013. https://doi.org/10.1007/978-3-319-48105-0_1 DOI: https://doi.org/10.1007/978-3-319-48105-0_1
A. Bhaduri, “Mechanical properties and working of metals and alloys,” Springer Series in Materials Science, 2018. https://doi.org/10.1007/978-981-10-7209-3 DOI: https://doi.org/10.1007/978-981-10-7209-3
P. Paris and F. Erdogan, “A critical analysis of crack propagation laws,” Journal of Basic Engineering, vol. 85, no. 4, pp. 528–533, 1963. https://doi.org/10.1115/1.3656900 DOI: https://doi.org/10.1115/1.3656900
R. Idris, S. Abdullah, P. Thamburaja, and M. Z. Omar, “Prediction of fatigue crack growth rate based on entropy generation,” Entropy, vol. 22, no. 1, p. 9, 2019. https://doi.org/10.3390/e22010009 DOI: https://doi.org/10.3390/e22010009
B. Hajshirmohammadi and M. M. Khonsari, “A simple approach for predicting fatigue crack propagation rate based on thermography,” Theoretical and Applied Fracture Mechanics, vol. 107, p. 102534, 2020. https://doi.org/10.1016/j.tafmec.2020.102534 DOI: https://doi.org/10.1016/j.tafmec.2020.102534
H. Salimi, M. Pourgol-Mohammad, and M. Yazdani, “Metal fatigue assessment based on temperature evolution and thermodynamic entropy generation,” International Journal of Fatigue, vol. 127, pp. 403–416, 2019. https://doi.org/10.1016/j.ijfatigue.2019.06.022 DOI: https://doi.org/10.1016/j.ijfatigue.2019.06.022
J. J. R. Faria, L. G. A. Fonseca, A. R. de Faria, A. Cantisano, T. N. Cunha, H. Jahed, and J. Montesano, “Determination of the fatigue behavior of mechanical components through infrared thermography,” Engineering Failure Analysis, vol. 134, p. 106018, 2022. http://dx.doi.org/10.1016/j.engfailanal.2021.106018 DOI: https://doi.org/10.1016/j.engfailanal.2021.106018
R. Cappello, G. Meneghetti, M. Ricotta, and G. Pitarresi, “On the correlation of temperature harmonic content with energy dissipation in C45 steel samples under fatigue loading,” Mechanics of Materials, vol. 168, p. 104271, 2022. https://doi.org/10.3221/IGF-ESIS.49.09 DOI: https://doi.org/10.1016/j.mechmat.2022.104271
A. Sendrowicz, A. O. Myhre, S. W. Wierdak, and A. Vinogradov, “Challenges and accomplishments in mechanical testing instrumented by in situ techniques: Infrared thermography, digital image correlation, and acoustic emission,” Applied Sciences, vol. 11, no. 15, p. 6718, 2021. https://doi.org/10.3390/app11156718 DOI: https://doi.org/10.3390/app11156718
G. Meneghetti and M. Ricotta, “Estimating the intrinsic dissipation using the second harmonic of the temperature signal in tension‐compression fatigue. part II: Experiments,” Fatigue & Fracture of Engineering Materials & Structures, vol. 44, no. 8, pp. 2153–2167, 2021. https://doi.org/10.1111/ffe.13484 DOI: https://doi.org/10.1111/ffe.13484
G. Meneghetti, M. Ricotta, and G. Pitarresi, “On the relation between J-integral and heat energy dissipation at the crack tip in stainless steel specimens,” Frattura ed Integrità Strutturale, vol. 13, no. 49, pp. 82–96, 2019. https://doi.org/10.3221/IGF-ESIS.49.09 DOI: https://doi.org/10.3221/IGF-ESIS.49.09
K. N. Pandey and S. Chand, “Analysis of temperature distribution near the crack tip under constant amplitude loading,” Fatigue & Fracture of Engineering Materials and Structures, vol. 31, no. 5, pp. 316–326, 2008. https://doi.org/10.1111/j.1460-2695.2008.01218.x DOI: https://doi.org/10.1111/j.1460-2695.2008.01218.x
G. Meneghetti and M. Ricotta, “A heat energy dissipation approach to elastic-plastic fatigue crack propagation,” Theoretical and Applied Fracture Mechanics, vol. 105, p. 102405, 2020. https://doi.org/10.1016/j.tafmec.2019.102405 DOI: https://doi.org/10.1016/j.tafmec.2019.102405
B. Wisner, K. Mazur, and A. Kontsos, “The use of Nondestructive Evaluation Methods in fatigue: A Review,” Fatigue & Fracture of Engineering Materials & Structures, vol. 43, no. 5, pp. 859–878, 2020. https://doi.org/10.1111/ffe.13208 DOI: https://doi.org/10.1111/ffe.13208
B. Hajshirmohammadi and M. M. Khonsari, “Thermographic evaluation of metal crack propagation during cyclic loading,” Theoretical and Applied Fracture Mechanics, vol. 105, p. 102385, 2020. https://doi.org/10.1016/j.tafmec.2019.102385 DOI: https://doi.org/10.1016/j.tafmec.2019.102385
Z. Boussattine, N. Ranc, and T. Palin-Luc, “About the heat sources generated during fatigue crack growth: What consequences on the stress intensity factor?,” Theoretical and Applied Fracture Mechanics, vol. 109, p. 102704, 2020. https://doi.org/10.1016/j.tafmec.2020.102704 DOI: https://doi.org/10.1016/j.tafmec.2020.102704
R. Idris, S. Abdullah, P. Thamburaja, and M. Z. Omar, “Predicting fatigue crack growth rate under block spectrum loading based on temperature evolution using the degradation-entropy generation theorem,” International Journal of Fracture, vol. 228, no. 2, pp. 145–158, 2021. https://doi.org/10.1007/s10704-021-00525-y DOI: https://doi.org/10.1007/s10704-021-00525-y
B. Hajshirmohammadi and M. M. Khonsari, “On the entropy of Fatigue Crack propagation,” International Journal of Fatigue, vol. 133, p. 105413, 2020. https://doi.org/10.1016/j.ijfatigue.2019.105413 DOI: https://doi.org/10.1016/j.ijfatigue.2019.105413
ASTM E647-15. Test Method for Measurement of Fatigue Crack Growth Rates. 2015. https://www.astm.org/e0647-15.html
K. Parthiban, S. Mohan Kumar, A. Rajesh Kannan, N. Siva Shanmugam, and K. Sankaranarayanasamy, “Microstructure and fatigue behavior of spin-arc welded AISI C1018 Low Carbon Steel,” Proceedings of the Institution of Mechanical Engineers, Part E: Journal of Process Mechanical Engineering, vol. 235, no. 6, pp. 2035–2044, 2021. https://doi.org/10.1177/09544089211027786 DOI: https://doi.org/10.1177/09544089211027786
ASTM A29/A29M. Specification for General Requirements for Steel Bars, Carbon and Alloy, Hot-Wrought. 2015. https://www.astm.org/a0029_a0029m-16.html
ASM International, “ASM Handbook Volume 1: Properties and Selection: Irons, Steels, and High-Performance Alloys,” 10th ed.; ASM International: Materials Park, OH, USA, 1990. https://www.asminternational.org/home/-/journal_content/56/10192/06181G/PUBLICATION
ASM International, “ASM Handbook. Volume 19: Fatigue and Fracture”. Materials Park Ohio: ASM International, 1996. https://doi.org/10.31399/asm.hb.v19.9781627081931. DOI: https://doi.org/10.31399/asm.hb.v19.9781627081931
B. M. Schönbauer, M. Fitzka, U. Karr, and H. Mayer, “Variable amplitude very high cycle fatigue of 17-4PH steel with a stepwise S-N curve,” International Journal of Fatigue, vol. 142, p. 105963, 2021. https://doi.org/10.1016/j.ijfatigue.2020.105963 DOI: https://doi.org/10.1016/j.ijfatigue.2020.105963
H. A. Richard and M. Sander, “Fatigue crack growth under cyclic loading with constant amplitude,” Fatigue Crack Growth, pp. 113–151, 2016. http://dx.doi.org/10.1007/978-3-319-32534-7_4 DOI: https://doi.org/10.1007/978-3-319-32534-7_4
M. Mehdizadeh, A. Haghshenas, and M. M. Khonsari, “On the effect of internal friction on torsional and axial cyclic loading,” International Journal of Fatigue, vol. 145, p. 106113, 2021. https://doi.org/10.1016/j.ijfatigue.2020.106113 DOI: https://doi.org/10.1016/j.ijfatigue.2020.106113
P. Sivák and E. Ostertagová, “Evaluation of fatigue tests by means of mathematical statistics,” Procedia Engineering, vol. 48, pp. 636–642, 2012. https://doi.org/10.1016/j.proeng.2012.09.564 DOI: https://doi.org/10.1016/j.proeng.2012.09.564
H. Salimi, M. Pourgol-Mohammad, and M. Yazdani, “Low-cycle fatigue assessment of metallic materials based on thermodynamic entropy generation – methodology and model development,” International Journal of Fatigue, vol. 144, p. 106058, 2021. https://doi.org/10.1016/j.ijfatigue.2020.106058 DOI: https://doi.org/10.1016/j.ijfatigue.2020.106058
C. Basaran, “Entropy-based fatigue, fracture, failure prediction and Structural Health Monitoring,” Entropy, vol. 22, no. 10, p. 1178, 2020. https://doi.org/10.1007/s10704-021-00525-y DOI: https://doi.org/10.3390/e22101178
J. Y. Jang and M. M. Khonsari, “Experimentally validated thermodynamic theory of metal fatigue,” Mechanics of Materials, vol. 160, p. 103927, 2021. https://doi.org/10.1016/j.mechmat.2021.103927 DOI: https://doi.org/10.1016/j.mechmat.2021.103927
J. Liu and X. Liu, “An improved method for fatigue life prediction of metal materials based on thermodynamic entropy,” International Journal of Fatigue, vol. 170, p. 107546, 2023. https://doi.org/10.1016/j.ijfatigue.2023.107546 DOI: https://doi.org/10.1016/j.ijfatigue.2023.107546
A. Nourian-Avval and M. M. Khonsari, “Rapid prediction of fatigue life based on thermodynamic entropy generation,” International Journal of Fatigue, vol. 145, p. 106105, 2021. https://doi.org/10.3390/e22101178 DOI: https://doi.org/10.1016/j.ijfatigue.2020.106105
M. Mehdizadeh, A. Haghshenas, and M. M. Khonsari, “In-situ technique for fatigue life prediction of metals based on temperature evolution,” International Journal of Mechanical Sciences, vol. 192, p. 106113, 2021. https://doi.org/10.1016/j.ijmecsci.2020.106113 DOI: https://doi.org/10.1016/j.ijmecsci.2020.106113
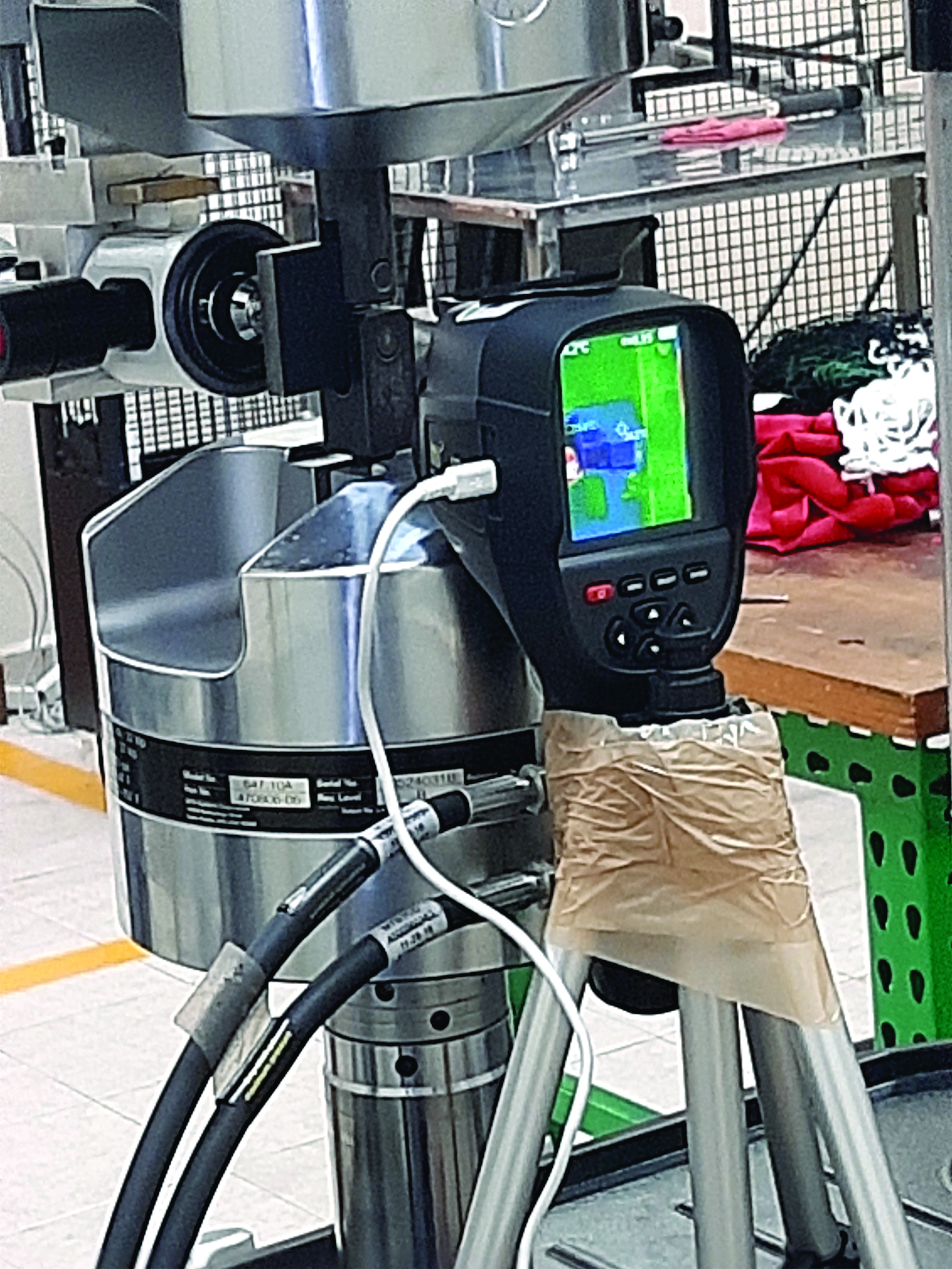
Published
How to Cite
Issue
Section
Categories
License
Copyright (c) 2023 Darío Antonio García Lavariega, Arturo Abúndez Pliego, Christian Jesús García López, Jan Mayén Chaires

This work is licensed under a Creative Commons Attribution 4.0 International License.
The authors who publish in this journal accept the following conditions:
The authors retain the copyright and assign to the journal the right of the first publication, with the work registered with the Creative Commons Attribution license 4.0, which allows third parties to use what is published as long as they mention the authorship of the work and the first publication in this magazine.
Authors may make other independent and additional contractual agreements for the non-exclusive distribution of the version of the article published in this journal (eg, include it in an institutional repository or publish it in a book) as long as they clearly indicate that the work it was first published in this magazine.
Authors are allowed and encouraged to share their work online (for example: in institutional repositories or personal web pages) before and during the manuscript submission process, as it can lead to productive exchanges, greater and more quick citation of published work (see The Effect of Open Access).