Modelado de sistemas termoeléctricos para la recolección energética en ambientes biológicos
DOI:
https://doi.org/10.37636/recit.v6n4e323Palabras clave:
Biosensores, Análisis de elemento finito, Bioenergía, Termogeneradores de energía, Simulación FEMResumen
En los últimos años la tendencia en el desarrollo emergente de biosensores autónomos y portátiles han propiciado la búsqueda de nuevos materiales, además del diseño de nuevas estructuras que generen su propia energía de manera eficiente y con alto rendimiento para asegurar el suministro energético a largo plazo, eliminando el uso de baterías externas. De los materiales más estudiados encontramos a los triboeléctricos, piezoeléctricos, termoeléctricos y piroeléctricos, materiales que han tenido mayor biocompatibilidad con los parámetros fisiológicos del cuerpo humano para la generación de energía. En este trabajo se propone ilustrar el proceso sistemático en un software de simulación de elementos finitos del material triteleluro de dibismuto (Bi2Te3), considerado como uno de los más eficientes en la generación de energía termoeléctrica. Las simulaciones implementadas en COMSOL MultiphysicsⓇ, demuestran la correlación entre el diseño físico-mecánico de las estructuras y la eficiencia energética, permitiendo la determinación de las características y parámetros esenciales para su futura fabricación. Los resultados demuestran que, con un número mayor de termopares, incluso cuando las dimensiones son inferiores tomando como referencia la temperatura superficial del cuerpo humano, aumenta la potencia generada de las estructuras evaluadas.
Descargas
Citas
S. Mondal, A. Dalvi, A. K. Mondal, and V. Thapa, "A novel approach for aerogenerators-triple state absorption-based cooling system", in Materials Today: Proceedings, May 2023. https://doi.org/10. 1016/j.matpr.2023.05.036 DOI: https://doi.org/10.1016/j.matpr.2023.05.036
M. Temiz, and I. Dincer, "Design and analysis of a floating photovoltaic based energy system with underground energy storage options for remote communities", J. Energy Storage, vol. 55, pt. C, Nov. 2022, Art. no. 105733. https://doi.org/10.1016/j.est. 2022.105733 DOI: https://doi.org/10.1016/j.est.2022.105733
C. Pestana Barros, "Efficiency analysis of hydroelectric generating plants: A case study for Portugal", Energy Econ., vol. 30, no. 1, pp. 59-75, Jan. 2008. https://doi.org/10.1016/j.eneco.2006.10. 008 DOI: https://doi.org/10.1016/j.eneco.2006.10.008
Q. Zhang, C. Xig, F. Shen, Y. Gong, Y. Zi, H. Gou, Z. Li, Y. Peng, Q. Zhang, and Z. L. Wang, "Human body IoT systems based on the triboelectrification effect: energy harvesting, sensing, interfacing and communication", Energy Environ. Sci., vol. 15, no. 9, pp. 3688-3721, Jul. 2022. https://doi.org/10.1039/D2EE01590K DOI: https://doi.org/10.1039/D2EE01590K
T. Tat, A. Libanori, C. Au, A. Yau, and J. Chen, "Advances in triboelectric nanogenerators for biomedical sensing," Biosens Bioelectron, vol. 171, Art. no. 112714, Jan. 2021. https://doi.org/10.1016 /j.bios.2020.112714 DOI: https://doi.org/10.1016/j.bios.2020.112714
F. Zamanpour, L. Shooshtari, M. Gholami, R. Mohammadpour, P. Sasanpour, and N. Taghavinia, "Transparent and flexible touch on/off switch based on BaTiO3/silicone polymer triboelectric nanogenerator", Nano Energy, vol. 103, pt. A, Art. no. 107796, Dec. 2022. https://doi.org/10.1016/j.na noen.2022.107796 DOI: https://doi.org/10.1016/j.nanoen.2022.107796
. X. Cheng, X. Xue, Y. Ma, M. Han, W. Zhang, Z. Xu, H. Zhang, and H. Zhang, "Implantable and self-powered blood pressure monitoring based on a piezoelectric thin-film: Simulated, in vitro and in vivo studies", Nano Energy, vol. 22, pp. 453-460, April 2016. https://doi.org/10.1016/j.nanoen.2016.02.037 DOI: https://doi.org/10.1016/j.nanoen.2016.02.037
. B. H. Moghadam, M. Hasanzadeh, and A. Simchi, "Self-Powered Wearable Piezoelectric Sensors Based on Polymer Nanofiber-Metal-Organic Framework Nanoparticle Composites for Arterial Pulse Monitoring", ACS Appl Nano Mater, vol. 3, no. 9, pp. 8742-8752, Aug. 2020. https://doi.org /10.1021/acsanm.0c01551 DOI: https://doi.org/10.1021/acsanm.0c01551
. Y. Wang, W. Zhu, Y. Deng, B. Fu, P. Zhu, Y. Yu, Jiao Li, and J. Guo, "Self-powered wearable pressure sensing system for continuous healthcare monitoring enabled by flexible thin-film thermoelectric generator", Nano Energy, vol. 73, Art. no. 104773, July 2020. https://doi.org/10.1016/j.nanoen.2020.10 4773 DOI: https://doi.org/10.1016/j.nanoen.2020.104773
. K. Meng, S. Zhao, Y. Zhou, Y. Wu, S. Zhang, Q. He, X. Wang, Z. Zhou, W. Fan, X. Tan, J. Yang, and J. Chen, "A Wireless Textile-Based Sensor System for Self-Powered Personalized Health Care", Matter, vol. 2, pp. 896-907, April 2020. https://doi.org/10.1016/j.matt.2019.12.025 DOI: https://doi.org/10.1016/j.matt.2019.12.025
P. Jha, P. Patra, J. Naik, A. Dutta, A. Acharya, P. Rajalakshmi, and S. G. Singh, "A 2μW biomedical frontend with ΣΔ ADC for self-powered U-healthcare devices in 0.18μm CMOS technology", en 2015 IEEE 13th International New Circuits and Systems Conference (NEWCAS), Grenoble, Francia, June 2015.https://doi.org/10.1109/NEWCAS.2015.7182054 DOI: https://doi.org/10.1109/NEWCAS.2015.7182054
M. L. S. Mi, S. H. M. Ali, and M. S. Islam, "A Novel Architecture of Maximum Power Point Tracking for Ultra-Low-Power Based Hybrid Energy Harvester in Ubiquitous Devices: A Review", Am. J. Appl. Sci., vol. 10, no. 10, pp. 1240-1251, Sep. 2013. https://doi.org/10.3844/ajassp.2013.1240.1251 DOI: https://doi.org/10.3844/ajassp.2013.1240.1251
. R. Kumar, F. J. Montero, R. Lamba, M. Vashishtha, and S. Upadhyaya, "Thermal management of photovoltaic-thermoelectric generator hybrid system using radiative cooling and heat pipe", Appl. Therm. Eng., vol. 227, Art. no. 120420, June 2023. https://doi.org/10.1016/j.applt hermaleng.2023.120420 DOI: https://doi.org/10.1016/j.applthermaleng.2023.120420
. Y. Zhu, D. W. Newbrook, C. H. de Groot, and R. Huang, "Comprehensive analysis of radiative cooling enabled thermoelectric energy harvesting", J. Phys. Photonics, vol. 5, no. 2, Art. no. 025002, April 2023. https://doi.org/10.1088/2515-7647/accac1 DOI: https://doi.org/10.1088/2515-7647/accac1
. F. J. Montero, R. Lamba, A. Ortega, W. Jahn, W. H. Chen, and A. M. Guzmán, "A bidirectional solar thermoelectric generator combining heat storage for daytime and nighttime power generation", Appl. Therm. Eng, vol. 224, Art. no. 119997, April 2023. https://doi.org/10.1016/j.applthermaleng.2023.119997 DOI: https://doi.org/10.1016/j.applthermaleng.2023.119997
. I. Vijitha, N. Jacob, N. Raveendran, C. Vijayakumar, and B. Deb, "Simulation-aided studies on the superior thermoelectric performance of printable PBDTT-FTTE/SWCNT composites", Mater. Today, Energy, vol. 32, art. no. 101233, March 2023. https://doi.org/10.1016/j.mtener.2022.101233 DOI: https://doi.org/10.1016/j.mtener.2022.101233
. Z. Varga, and E. Racz, "COMSOL 3D Simulation of Optimization of Waste Heat Recovery Using Thermoelectric Generator by Modifying the Design", 2022 IEEE 5th International Conference and Workshop in Obuda on Electrical and Power Engineering (CANDO-EPE), Budapest, Hungria, pp. 000101-000106, 2022. https://doi.org/10.1109/CAN DOEPE57516.2022.10046369 DOI: https://doi.org/10.1109/CANDO-EPE57516.2022.10046369
T. Kucova, P. Prauzek, J. Konecny, M. Zilys, and R. Martinek, "Thermoelectric energy harvesting for internet of things devices using machine learning: A review", CAAI Trans Intell Technol, pp. 1-21, July 2023. https://doi.org/10.1049/cit2.12259 DOI: https://doi.org/10.1049/cit2.12259
Y. Hou, Y. Yang, Z. Wang, Z. Li, X. Zhang, B. Bethers, R. Xiong, H. Guo, and H. Yu, "Whole fabric-assisted thermoelectric devices for wearable electronics", Adv. Sci., vol. 9, no. 1, Art. no. 2103574, Nov. 2022. https://doi.org/10.1002/advs. 202103574 DOI: https://doi.org/10.1002/advs.202270002
Y. Wang, Y. Shi, D. Mei, and Z. Chen, "Wearable thermoelectric generator to harvest body heat for powering a miniaturized accelerometer". Appl. Energy, vol. 215, pp. 690-698, April 2018. https://doi.org/10.1016/j.apenergy.2018.02.062 DOI: https://doi.org/10.1016/j.apenergy.2018.02.062
M. Dargusch, W. D. Liu, and Z. G. Chen, "Thermoelectric generators: alternative power supply for wearable electrocardiographic systems". Adv Sci, vol. 7, no. 18, Art. no. 2001362, Aug. 2020. https://doi.org/10.1002/advs.202001362 DOI: https://doi.org/10.1002/advs.202001362
S. Addanki, and D. Nedumaran, "Simulation and fabrication of thermoelectric generators for hand held electronic gadgets", Mater Sci Engineering: B, vol. 251, Art. no. 114453, Dec. 2019. https://doi.org /10.1016/j.mseb.2019.114453 DOI: https://doi.org/10.1016/j.mseb.2019.114453
C. Rudderham, and J. Maassen, "Ab initio thermoelectric calculations of ring-shaped bands in two-dimensional Bi2Te3, Bi2Se3, and Sb2Te3: Comparison of scattering approximations", Physical Rev B, vol. 103, n. 16, April 2021. https://doi.org/10.1103/PhysRevB.103.165406 DOI: https://doi.org/10.1103/PhysRevB.103.165406
L. Yi, H. Xu, H. Yang, S. Huang, H. Yang, Y. Li, Q. Zhang, Z. Guo, H. Hu, P. Sun, X. Tan, G. Liu, K. Song, and J. Jiang, "Design of Bi2te3-Based Thermoelectric Generator in a Widely Applicable System", J. Power Sources, vol. 559, March 2022. https://doi.org/10.2139/ssrn.4218766 DOI: https://doi.org/10.2139/ssrn.4218766
W. Glatz, E. Schwyter, L. Durrer, and C. Hierold, "Bi2Te3-Based Flexible Micro Thermoelectric Generator with Optimized Design", J. Microelectromech Syst, vol. 18, n. 3, pp. 763-772, June 2009. https://doi.org/10.1109/JMEMS.2009.202 1104 DOI: https://doi.org/10.1109/JMEMS.2009.2021104
Ho Sung Lee, "Thermoelectric Generators", in Thermoelectrics: Design and Materials, Chichester, UK: John Wiley & Sons, pp. 8-22, 2016. https://doi.org/10.1002/9781118848944 DOI: https://doi.org/10.1002/9781118848944.ch2
J. Garcia-Cañadas, "Thermoelectric Modules: Power Output, Efficiency, and characterization", in Thermoelectric Energy Conversion: Basic Concepts and Device Applications, D. D. Pineda y A. Rezaniakolaei, Eds., Dinamarca, Wiley-VCH Verlag GmbH y Co. KGaA., pp. 127-146, 2017. https://doi.org/10.1002/9783527698110.ch7 DOI: https://doi.org/10.1002/9783527698110.ch7
The Heat Transfer Module, User's Guide, COMSOL 5.4. Available: https://doc.comsol.com /5.4/doc/com.comsol.help.heat/HeatTransferModuleUsersGuide.pdf. [Accessed: 03, Aug. 2023]
AC/DC Module, User's Guide, COMSOL 5.4. Available:https://doc.comsol.com/5.4/doc/com.comsol.help.acdc/ACDCModuleUsersGuide.pdf. [Accessed: 03, Aug. 2023]
The Finite Element Method (FEM). “An Introduction to the Finite Element Method”, COMSOL, 2017. available: https://www.comsol.com /multiphysics/finite-element-method. [Accessed: 29, July, 2023]
J. L. Perez-Aparicio, R. L. Taylor, and D. Gavela, "Finite element analysis of nonlinear fully coupled thermoelectric materials", Comput Mech, vol. 40, pp. 35-45, June 2007. https://doi.org/10.10 07/s00466-006-0080-7 DOI: https://doi.org/10.1007/s00466-006-0080-7
W. Glatz, S. Muntwyler, and C. Hierold, "Optimization and fabrication of thick flexible polymer-based micro thermoelectric generator," Sens Actuators A, Phys, vol. 132, no. 1, pp. 337-345, Nov. 2006. https://doi.org/10.1016/j.sna.2006.04.024 DOI: https://doi.org/10.1016/j.sna.2006.04.024
R. Ahmed, Zeeshan, M. U. Mehmood, A. Mannan, J. Y. Lee, S. H. Lim, and W. Chun, "Design and performance analysis of powering a wireless earphone by a thermoelectric generator", IEEE Access, vol. 9, pp. 54457-54465, Feb. 2021. https://doi.org/10.1109/ACCESS.2021.3062086 DOI: https://doi.org/10.1109/ACCESS.2021.3062086
M. K. Kim, M. S. Kim, S. Lee, C. Kim, and Y. J. Kim. "Wearable thermoelectric generator for harvesting human body heat energy", Smart Mater Struct, vol. 23, no. 10, Art. no. 105002, Sep. 2014. https://doi.org/10.1088/0964-1726/23/10/105002 DOI: https://doi.org/10.1088/0964-1726/23/10/105002
T. Sugahara, Y. Ekubaru, N. V. Nong, N. Kagami, K. Ohata, L. T. Hung, M. Okajima, S. Nambu, and K. Suganuma, "Fabrication with semiconductor packaging technologies and characterization of a large-scale flexible thermoelectric module", Adv Mater, Technol, vol. 4, no. 2, art. no. 1800556, Dec. 2018. https://doi.org/10.1088/0964-1726/23/10/105002 DOI: https://doi.org/10.1002/admt.201800556
B. Lee, H. Cho, K. T. Park, J. S. Kim, M. Park, H. Kim, Y. Hong, and S. Chung, "High-performance compliant thermoelectric generators with magnetically self-assembled soft heat conductors for self-powered wearable electronics", Nat. Commun., vol. 11, art. no. 5948, Nov. 2020. https://doi.org/10.1038/s41467-020-19756-z DOI: https://doi.org/10.1038/s41467-020-19756-z
C. S. Kim, H. M. Yang, J. Lee, G. S. Lee, H. Choi, Y. Kim, S. H. Lim, S. H. Cho, and B. J. Cho, "Self-powered wearable electrocardiography using a wearable thermoelectric power generator", ACS Energy Lett., vol. 3, no. 3, pp. 501-507, Jan. 2018. https://doi.org/10.1021/acsenergylett.7b01237 DOI: https://doi.org/10.1021/acsenergylett.7b01237
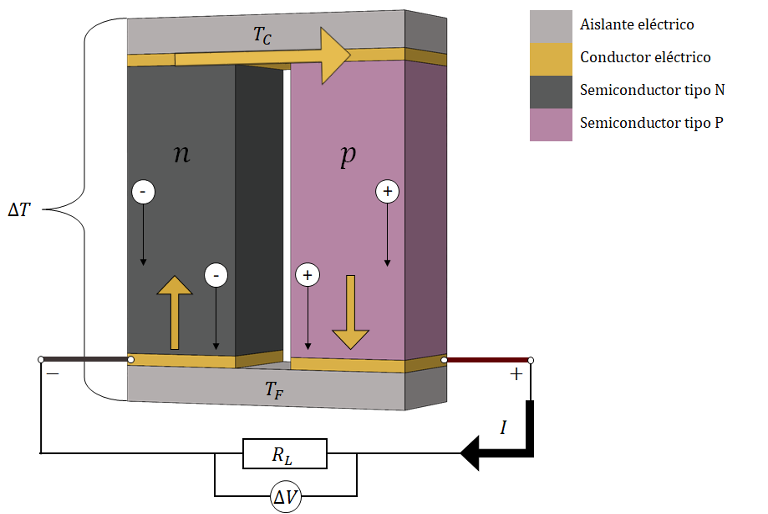
Publicado
Cómo citar
Número
Sección
Categorías
Licencia
Derechos de autor 2023 Martha Alexandra Gómez Caraveo, Sharon Ezrre González, José Alejandro Amézquita García, Heriberto Márquez Becerra

Esta obra está bajo una licencia internacional Creative Commons Atribución 4.0.
Los autores/as que publiquen en esta revista aceptan las siguientes condiciones:
- Los autores/as conservan los derechos de autor y ceden a la revista el derecho de la primera publicación, con el trabajo registrado con la licencia de atribución de Creative Commons 4.0, que permite a terceros utilizar lo publicado siempre que mencionen la autoría del trabajo y a la primera publicación en esta revista.
- Los autores/as pueden realizar otros acuerdos contractuales independientes y adicionales para la distribución no exclusiva de la versión del artículo publicado en esta revista (p. ej., incluirlo en un repositorio institucional o publicarlo en un libro) siempre que indiquen claramente que el trabajo se publicó por primera vez en esta revista.
- Se permite y recomienda a los autores/as a compartir su trabajo en línea (por ejemplo: en repositorios institucionales o páginas web personales) antes y durante el proceso de envío del manuscrito, ya que puede conducir a intercambios productivos, a una mayor y más rápida citación del trabajo publicado (vea The Effect of Open Access).