Procesamiento de polvos de colector de alto horno para su aprovechamiento en la industria siderúrgica
DOI:
https://doi.org/10.37636/recit.v7n3e277Palabras clave:
Alto horno, Polvo de colector, Lixiviación, Separación magnética, Procesamiento de polvosResumen
La producción de arrabio implica la generación de subproductos como los polvos de colector, que son atractivos debido a su contenido de Fe, sin embargo, no pueden reutilizarse directamente debido a su alto contenido de Zn y álcalis. Por ello, esta investigación, busca su aprovechamiento, mediante lixiviación ácida. Para esto, una muestra fue procesada mediante molienda (1h) y separación magnética de baja intensidad y luego se evaluó el efecto del ácido clorhídrico como agente lixiviante en cada etapa del proceso (0.10, 0.25, 0.50 y 1.0M HCl). Además, se analizó el efecto de la temperatura para el sistema con 1M HCl. Los resultados indican que al trabajar en los sistemas con alta concentración de HCl tanto en el material fresco como el molido, fue posible disolver el contenido de Zn hasta un 65%. Por otro lado, al lixiviar los polvos tratados por separación magnética, se alcanzaron disoluciones de Zn de 56 y 65% para 1 y 2 limpias respectivamente. En cuanto al contenido de Fe, se tuvo un incremento respecto al contenido inicial, pasando de 53.8 a 59% Fe, (lo cual, hace factible su procesamiento). Por otra parte, a medida que aumentó la temperatura, se favoreció la lixiviación de Zn, a 85°C, se alcanzó hasta un 74% de disolución. En el caso de la disolución de Na2O, P y K2O el incremento en la temperatura de trabajo acelera la cinética de disolución, no obstante, en el caso de Fe, el incremento en la temperatura de trabajo, provoca una disminución en su concentración. Por último, en el análisis termodinámico se determinaron los valores de ∆G° de las reacciones, indicando que son espontáneas, es decir, que se llevan a cabo sin la necesidad de una energía aplicada. En conclusión, fue posible comparar el efecto de los sistemas de lixiviación en cada etapa del procesamiento. A temperatura ambiente, la lixiviación del polvo sin procesar alcanza hasta 65% de disolución de Zn con 38.8% Fe; y luego de 2 limpias magnéticas, se alcanza una disolución de 65% Zn con contenido de 61.4% Fe. Al incrementar la temperatura, se cataliza la mayoría de las reacciones involucradas en el proceso de lixiviación, especialmente la de disolución de zinc (hasta 74%) y mediante el uso de la termodinámica se puede sustentar la factibilidad de las reacciones involucradas.
Descargas
Citas
I. Cameron, L. Sukhram, K. Lefebvre and W. Davenport, Blast Furnace Ironmaking: Analysis, Control, and Optimization, (Edition unavailable), Elsevier Science, 2019. [E-book] Available: https://doi.org/10.1016/B978-0-12-814227-1.00061-0 DOI: https://doi.org/10.1016/B978-0-12-814227-1.00061-0
P. Li, G. Wang, Y. Dong and Y. Zhuo, “A Review on Desulfurization Technologies of Blast Furnace Gases,” Curr. Pollut. Rep., vol. 8, no. 2, pp. 189–200, 2022. https://doi.org/10.1007/s40726-022-00212-z DOI: https://doi.org/10.1007/s40726-022-00212-z
M. Oge, D. Ozkan, M. B. Celik, M. S. Gok and A. C. Karaoglanli, “An Overview of Utilization of Blast Furnace and Steelmaking Slag in Various Applications”, Mater. Today: Proc., vol. 11, no. 1, pp. 516-525, 2019. https://doi.org/10.1016/j.matpr.2019.01.023. DOI: https://doi.org/10.1016/j.matpr.2019.01.023
C. Hamann, M. Spanka, D. Stolle, G. Auer, E. Weingart, D. Al-Sabbagh, M. Ostermann and C. Adam, “Recycling of blast-furnace sludge by thermochemical treatment with spent iron(II) chloride solution from steel pickling,” J. Hazard. Mater., vol. 402, 2020. https://doi.org/10.1016/j.jhazmat.2020.123511 DOI: https://doi.org/10.1016/j.jhazmat.2020.123511
R. Ochoa and M. Farfan, “Blast furnace dust: An alternative for the improvement of granular material for pavements. J. Phys. Conf. Ser., vol.1386, 2019. https://doi.org/10.1088/1742-6596/1386/1/012033 DOI: https://doi.org/10.1088/1742-6596/1386/1/012033
X. Yang, B. Xie, F. Wang, P. Ning, K. Li, L. Jia, J. Feng, F. Xia, “Resource utilization of hazardous solid waste blast furnace dust: Efficient wet desulfurization and metal recovery,” Chemosphere, vol. 314, pp. 137592. 2023. https://doi.org/10.1016/j.chemosphere.2022.137592 DOI: https://doi.org/10.1016/j.chemosphere.2022.137592
Y. Takahashi and S. Suntharalingam, “Experimental Study on Autogenous Shrinkage Behaviors of Different Portland Blast Furnace Slag Cements,” Constr. Build. Mater., vol. 230, 2020. https://doi.org/10.1016/j.conbuildmat.2019.116980 DOI: https://doi.org/10.1016/j.conbuildmat.2019.116980
L. Ding, W Ning, Q. Wang, D. Shi and L. Luo, “Preparation and characterization of glass-ceramic foams from blast furnace slag and waste glass,” Mater. Lett., vol. 141, pp. 327-329, 2015. https://doi.org/10.1016/j.matlet.2014.11.122 DOI: https://doi.org/10.1016/j.matlet.2014.11.122
H. A. Rondón, J. C. Ruge, D. F. Patiño, H. A. Vacca, F. A. Reyes and M. Muniz, “Blast furnace slag as a substitute for the fine fraction of aggregates in an asphalt mixture,” J. Mater. Civ. Eng., vol. 30, no. 10, 2018. https://doi.org/10.1061/(ASCE)MT.1943-5533.0002409 DOI: https://doi.org/10.1061/(ASCE)MT.1943-5533.0002409
R. Mahakhud, M. Priyadarshini and J. Prakash, “Utilization of ground granulated blast-furnace slag powder in brick industry: A new generation building material,” Mater. Today: Proc., 2023. https://doi.org/10.1016/j.matpr.2023.03.707 DOI: https://doi.org/10.1016/j.matpr.2023.03.707
X. Yin, C. M. Zhang, Y. H. Cai, C. M. Zhao, J. Yang and B. Li, “Molten slag bath reduction: Carbon-thermal reduction of blast furnace dust in molten blast furnace slag,” IOP Conf. Ser.: Mater. Sci. Eng., vol. 230, no. 1, pp. 012027, 2017. https://dx.doi.org/10.1088/1757-899X/230/1/012027 DOI: https://doi.org/10.1088/1757-899X/230/1/012027
G. Zhao, R. Li, X. Xing, J. Ju, X. Li, J. Zu, “Removal behavior of Zn and alkalis from blast furnace dust in pre-reduction sinter process,” Green Process. Synth., vol.12, no. 1, pp. 20230045, 2023. https://doi.org/10.1515/gps-2023-0045 DOI: https://doi.org/10.1515/gps-2023-0045
X. Liu, Z. Liu, J. Zhang and X. Xing, "Recovery of Iron and Zinc from Blast Furnace Dust Using Iron-Bath Reduction," High Temp. Mater. Process., vol. 38, no. 2019, pp. 767-772, 2019. https://doi.org/10.1515/htmp-2019-0023 DOI: https://doi.org/10.1515/htmp-2019-0023
S. Mustafa, L. Luo, B.-T. Zheng, C.-X. Wei and N. Christophe, “Effect of Lead and Zinc Impurities in Ironmaking and the Corresponding Removal Methods: A Review,” Metals, vol. 11, no. 3, pp. 407, 2021. https://doi.org/10.3390/met11030407 DOI: https://doi.org/10.3390/met11030407
A. Andersson, H. Ahmed, J. Rosenkranz, C. Samuelsson and B. Bjorkman, “Characterization and upgrading of a low Zinc-containing and fine blast furnace sludge – a multi objective analysis,” ISIJ Int., vol. 57, no. 2, pp. 262-271, 2017. https://doi.org/10.2355/isijinternational.ISIJINT-2016-512 DOI: https://doi.org/10.2355/isijinternational.ISIJINT-2016-512
I. O. Acuña, J. A. López and A. Perea, “Lixiviación de Zn a partir de polvo del colector del alto horno para su aplicación potencial como material reciclado,” CienciAcierta Revista científica, tecnológica y humanística, no. 62, 2020.
N. Nayak, “Characterization of blast furnace flue dust- an assessment for its utilization,” Mater. Today: Proc., vol.50, no. 2, pp. 2078-2083, 2021. https://doi.org/10.1016/j.matpr.2021.09.417 DOI: https://doi.org/10.1016/j.matpr.2021.09.417
K. Brunelli and M. Dabalá, “Ultrasound effects on zinc recovery from EAF dust by sulfuric acid leaching,” Int. J. Miner., Metall. Mater., vol. 22, no. 4, pp. 353-362, 2015. https://doi.org/10.1007/s12613-015-1080-4 DOI: https://doi.org/10.1007/s12613-015-1080-4
L. Hernández, I. Daza, G. Amaral, F. Beneduce and G. Lenz, “Microstructural, thermochemistry and mechanical evaluation of self-reducing pellets using electric arc furnace (EAF) dust containing zinc for Waelz process,” Matéria, vol. 23, no. 2, 2018. https://doi.org/10.1590/S1517-707620180002.0343 DOI: https://doi.org/10.1590/s1517-707620180002.0343
P. Dvorak and H. Vu, “Zinc recovery from flue dust,” Journal of the polish mineral engineering society, vol. 18, pp. 195-198, 2017. https://doi.org/10.29227/IM-2017-01-31
Y. Xue, X. Hao, X. Liu, and N. Zhang, “Recovery of Zinc and Iron from Steel Mill Dust—An Overview of Available Technologies,” Materials, vol. 15, no. 12, pp. 4127, 2022. https://doi.org/10.3390/ma15124127 DOI: https://doi.org/10.3390/ma15124127
H. S. Lee, D. Park, Y. Hwang, J. Ha, H. Shin, “Toward high recovery and selective leaching of zinc from electric arc furnace dust with different physicochemical properties,” Environ. Eng. Res., vol. 25, no. 3, pp. 335-344, 2019. https://doi.org/10.4491/eer.2019.132 DOI: https://doi.org/10.4491/eer.2019.132
K. Binnemans, P. T. Jones, P.T., A. Fernández and V. Torres “Hydrometallurgical Processes for the Recovery of Metals from Steel Industry By-Products: A Critical Review,” J. Sustain. Metall., vol. 6, pp. 505–540 (2020). https://doi.org/10.1007/s40831-020-00306-2 DOI: https://doi.org/10.1007/s40831-020-00306-2
F. Kukurugya, T. Vindt, and T. Havlík, “Behavior of zinc, iron and calcium from electric arc furnace (EAF) dust in hydrometallurgical processing in sulfuric acid solutions: Thermodynamic and kinetic aspects,” Hydrometallurgy, vol. 154, pp. 20–32, 2015. https://doi.org/10.1016/j.hydromet.2015.03.008 DOI: https://doi.org/10.1016/j.hydromet.2015.03.008
R. Kusumaningrum, A. Fitroturokhmah, G. Sinaga, A. Wismogroho, W. Widayatno, L. J. Diguna and Amal, Muhamad, “Study: leaching of zinc dust from electric arc furnace waste using oxalic acid,” IOP Conf. Ser. Mater. Sci. Eng., vol. 478, no. 2019, pp. 012015, 2019. https://doi.org/10.1088/1757-899X/478/1/012015 DOI: https://doi.org/10.1088/1757-899X/478/1/012015
M. J. Soria, G. I. Davila, F. R. Carrillo, A. A. Gonzalez, N. Picazo, F. J. Lopez, J. Ramos, “Oxidative Leaching of Zinc and Alkalis from Iron Blast Furnace Sludge,” Metals, vol. 9, no. 9, pp. 1015, 2019. https://doi.org/10.3390/met9091015 DOI: https://doi.org/10.3390/met9091015
Y. Y. Teo, H. S. Lee, Y. C. Low, S. W. Choong and K. O. Low, “Hydrometallurgical extraction of zinc and iron from electric arc furnace dust (EAFD) using hydrochloric acid,” J. Phys. Sci., vol. 29, no. 3, pp. 49–54, 2018. https://doi.org/10.21315/jps2018.29.s3.6
S. Langová, J. Lesko and D. Matysek, “Selective leaching of zinc from zinc ferrite with hydrochloric acid,” Hydrometallurgy, vol. 95, no. 179-182, 2009. https://doi.org/10.1016/j.hydromet.2008.05.040 DOI: https://doi.org/10.1016/j.hydromet.2008.05.040
M. Irannajad, M. Meshkini, A. R. Azadmehr, “Leaching of zinc from low grade oxide ore using organic ACID,” Physicochem. Probl. Miner. Process., vol. 49, no. 2, pp. 547-555, 2013. https://doi.org/10.21315/jps2018.29.s3.6 DOI: https://doi.org/10.21315/jps2018.29.s3.6
J. Borda and R. Torres, “Comparative study of selective zinc leaching from EAFD using carboxylic agents,” Rev. Mex. Ing. Quím., vol. 20, no. 1, 2021. https://doi.org/10.24275/rmiq/IA2022 DOI: https://doi.org/10.24275/rmiq/IA2022
R. Larba, I. Boukerche, N. Alane, N. Habbache, S. Djerad and L. Tifouti, “Citric acid as an alternative lixiviant for zinc oxide dissolution,” Hydrometallurgy, vol. 134, pp. 117–123, 2013. https://doi.org/10.1016/j.hydromet.2013.02.002 DOI: https://doi.org/10.1016/j.hydromet.2013.02.002
R. Baidya, S. Kumar and U. Parlikar, “Blast furnace flue dust co-processing in cement kiln - A pilot study,” Waste Manag. Res., vol. 37, no. 3, pp. 261–267, 2019. https://doi.org/10.1177/0734242X18816791 DOI: https://doi.org/10.1177/0734242X18816791
B. Das, S. Prakash, P. Reddy, S. Biswal, B. Mohapatra and V. Misra, “Effective utilization of blast furnace flue dust of integrated steel plants,” The European Journal of Mineral Processing and Environmental Protection, vol. 2, no. 2, pp. 61-68, 2002.
A. Yehia and F. El-Rehiem, “Recovery and utilization of iron and carbon values from blast furnace flue dust,” Miner. Process. Extr. Metall., vol. 114, no. 4, pp. 207-211, 2005. https://doi.org/10.1179/037195505X28519 DOI: https://doi.org/10.1179/037195505X28519
J. Ju, Y. Feng, H. Li, Q. Zhang, “Study of recycling blast furnace dust by magnetization roasting with straw charcoal as reductant,” Physicochem. Probl. Miner. Process., vol. 58, no. 3, pp. 149265, 2022. https://doi.org/10.37190/ppmp/149265 DOI: https://doi.org/10.37190/ppmp/149265
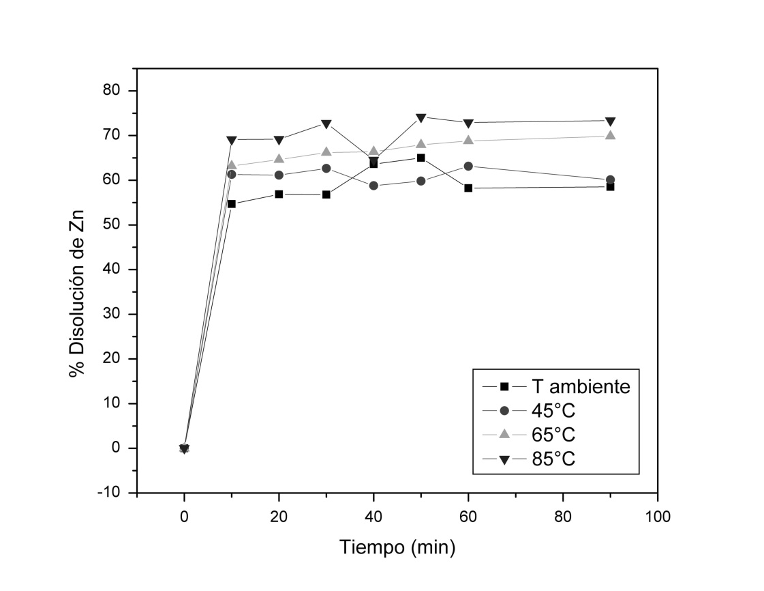
Publicado
Cómo citar
Número
Sección
Categorías
Licencia
Derechos de autor 2024 Iván Omar Acuña Gutiérrez, Damaris Margarita Puente Siller, José Manuel González de la Cruz, Luis Enrique Álvarez García, Juan Antonio López Corpus, Alberto Perea Garduño

Esta obra está bajo una licencia internacional Creative Commons Atribución 4.0.
Los autores/as que publiquen en esta revista aceptan las siguientes condiciones:
- Los autores/as conservan los derechos de autor y ceden a la revista el derecho de la primera publicación, con el trabajo registrado con la licencia de atribución de Creative Commons 4.0, que permite a terceros utilizar lo publicado siempre que mencionen la autoría del trabajo y a la primera publicación en esta revista.
- Los autores/as pueden realizar otros acuerdos contractuales independientes y adicionales para la distribución no exclusiva de la versión del artículo publicado en esta revista (p. ej., incluirlo en un repositorio institucional o publicarlo en un libro) siempre que indiquen claramente que el trabajo se publicó por primera vez en esta revista.
- Se permite y recomienda a los autores/as a compartir su trabajo en línea (por ejemplo: en repositorios institucionales o páginas web personales) antes y durante el proceso de envío del manuscrito, ya que puede conducir a intercambios productivos, a una mayor y más rápida citación del trabajo publicado (vea The Effect of Open Access).