Polymeric membranes doped with copper nanoparticles synthesized by green chemistry using a saffron extract
DOI:
https://doi.org/10.37636/recit.v8n2e404Keywords:
Copper nanoparticles, Green chemistry, Copper-membrane nanocomposites, Fouling surfaceAbstract
Recently, interest in metal nanoparticles (MNPs) has increased significantly, due to the special properties and potential applications they present in diverse areas. Copper nanoparticles (CuNPs) in particular have attracted considerable attention due to their conductive, catalytic, optical, electrical and antibacterial properties. In the present research work, a methodology has been developed to support, by in situ and ex situ methods, CuNPs, synthesized by green chemistry using an aqueous extract of saffron (ExCs), on polymeric ultrafiltration membranes. The obtained nanocomposites (CuNPs/ExCs) were characterized by FTIR/ATR, field emission scanning electron microscopy (FESEM-EDS), XRD, thermogravimetric analysis (TGA), and permeability and fouling rate analysis. The permeability results obtained show that the method used to support the CuNPs/ExCs on the polymeric membrane, affects their permeability and fouling degree. The composites obtained using the in situ methodology (using sodium borohydride or ascorbic acid, as reducing agents), presented higher permeability (1.14x10-8 m/Pa.s and 1.94x10-9 m/Pa.s, respectively) and membrane surface fouling rates of 28.2 and 2.89%, respectively. The results demonstrate that CuNPs repel organic matter, thus reducing fouling of the polymeric membrane surface.
Downloads
References
[1] A. Arya, K. Gupta, T. S. Chundawat, and D. Vaya, “Biogenic synthesis of copper and silver nanoparticles using green alga Botryococcus braunii and its antimicrobial activity,” Bioinorg. Chem. Appl., vol. 2018, no. 1, p. 7879403, January 2018, https://doi.org/10.1155/2018/7879403. DOI: https://doi.org/10.1155/2018/7879403
[2] S. J. Ahmadi, M. Outokesh, M. Hosseinpour, and T. Mousavand, “A simple granulation technique for preparing high-porosity nano copper oxide(II) catalyst beads,” Particuology, vol. 9, no. 5, pp. 480–485, October 2011, https://doi.org/10.1016/j.partic.2011.02.010. DOI: https://doi.org/10.1016/j.partic.2011.02.010
[3] J. Suárez-Cerda, H. Espinoza-Gómez, G. Alonso-Núñez, I. A. Rivero, Y. Gochi-Ponce, and L. Z. Flores-López, “A green synthesis of copper nanoparticles using native cyclodextrins as stabilizing agents,” J. Saudi Chem. Soc., vol. 21, no. 3, March 2017, https://doi.org/10.1016/j.jscs.2016.10.005. DOI: https://doi.org/10.1016/j.jscs.2016.10.005
[4] G. F. Galal, B. T. Abd-Elhalim, K. A. Abou-Taleb, A. A. Haroun, and R. F. Gamal, “Toxicity assessment of green synthesized Cu nanoparticles by cell-free extract of Pseudomonas silesiensis as antitumor cancer and antimicrobial,” Ann. Agric. Sci., vol. 66, no. 1, pp. 8–15, June 2021, https://doi.org/10.1016/j.aoas.2021.01.006. DOI: https://doi.org/10.1016/j.aoas.2021.01.006
[5] S. Adewale, A. Kolawole, S. Charles, and D. Felix, “Green chemistry approach towards the synthesis of copper nanoparticles and its potential applications as therapeutic agents and environmental control,” Curr. Res. Green Sustain. Chem., vol. 4, no. July, p. 100176, 2021, https://doi.org/10.1016/j.crgsc.2021.100176. DOI: https://doi.org/10.1016/j.crgsc.2021.100176
[6] S. A. Akintelu, A. S. Folorunso, F. A. Folorunso, and A. K. Oyebamiji, “Green synthesis of copper oxide nanoparticles for biomedical application and environmental remediation,” Heliyon, vol. 6, no. 7, p. e04508, July 2020, https://doi.org/10.1016/j.heliyon.2020.e04508. DOI: https://doi.org/10.1016/j.heliyon.2020.e04508
[7] A. Nieto-Maldonado et al., “Green synthesis of copper nanoparticles using different plant extracts and their antibacterial activity,” J. Environ. Chem. Eng., vol. 10, no. 2, April 2022, https://doi.org/10.1016/j.jece.2022.107130. DOI: https://doi.org/10.1016/j.jece.2022.107130
[8] V. Kumar, R. Saha, S. Chatterjee, and V. Mishra, “From traditional to greener alternatives: potential of plant resources as a biotransformation tool in organic synthesis,” React. Chem. Eng., vol. 8, no. 11, pp. 2677–2688, September 2023, https://doi.org/10.1039/D3RE00346A. DOI: https://doi.org/10.1039/D3RE00346A
[9] I. Jabeen et al., “Green synthesis and biological applications of Peganum harmala mediated copper oxide nanoparticles,” J. Mol. Struct., vol. 1325, no. October 2024, p. 140838, 2025, https://doi.org/10.1016/j.molstruc.2024.140838. DOI: https://doi.org/10.1016/j.molstruc.2024.140838
[10] M. Nasrollahzadeh and S. Mohammad Sajadi, “Green synthesis of copper nanoparticles using Ginkgo biloba L. leaf extract and their catalytic activity for the Huisgen [3+2] cycloaddition of azides and alkynes at room temperature,” J. Colloid Interface Sci., vol. 457, pp. 141–147, November 2015, https://doi.org/10.1016/j.jcis.2015.07.004. DOI: https://doi.org/10.1016/j.jcis.2015.07.004
[11] A. Thirumurugan, P. Aswitha, C. Kiruthika, S. Nagarajan, and A. N. Christy, “Green synthesis of platinum nanoparticles using Azadirachta indica- An eco-friendly approach,” Mater. Lett., vol. 170, pp. 175–178, May 2016, https://doi.org/10.1016/j.matlet.2016.02.026. DOI: https://doi.org/10.1016/j.matlet.2016.02.026
[12] N. Nagar and V. Devra, “Green synthesis and characterization of copper nanoparticles using Azadirachta indica leaves,” Mater. Chem. Phys., vol. 213, pp. 44–51, 2018, https://doi.org/10.1016/j.matchemphys.2018.04.007. DOI: https://doi.org/10.1016/j.matchemphys.2018.04.007
[13] Y. Wei, Z. Fang, L. Zheng, L. Tan, and E. P. Tsang, “Green synthesis of Fe nanoparticles using Citrus maxima peels aqueous extracts,” Mater. Lett., vol. 185, no. 9, pp. 384–386, December 2016, https://doi.org/10.1016/j.matlet.2016.09.029. DOI: https://doi.org/10.1016/j.matlet.2016.09.029
[14] S. Shende, A. P. Ingle, A. Gade, and M. Rai, “Green synthesis of copper nanoparticles by Citrus medica Linn. (Idilimbu) juice and its antimicrobial activity,” World J. Microbiol. Biotechnol., vol. 31, no. 6, pp. 865–873, March 2015, https://doi.org/10.1007/s11274-015-1840-3. DOI: https://doi.org/10.1007/s11274-015-1840-3
[15] P. Sharma, S. Pant, V. Dave, K. Tak, and V. Sadhu, “Green synthesis and characterization of copper nanoparticles by Tinospora cardifolia to produce nature-friendly copper nano-coated fabric and their antimicrobial evaluation,” J. Microbiol. Methods, vol. 160, no.1 , pp. 107–116, January 2019, https://doi.org/10.1016/j.mimet.2019.03.007. DOI: https://doi.org/10.1016/j.mimet.2019.03.007
[16] T. Abu-Izneid et al., “Nutritional and health beneficial properties of saffron (Crocus sativus L): a comprehensive review,” Crit. Rev. Food Sci. Nutr., vol. 62, no. 10, pp. 2683–2706, April 2022, https://doi.org/10.1080/10408398.2020.1857682. DOI: https://doi.org/10.1080/10408398.2020.1857682
[17] X. Li et al., “Stigma and petals of Crocus sativus L.: Review and comparison of phytochemistry and pharmacology,” Arab. J. Chem., p. 104918, August 2023, https://doi.org/10.1016/j.arabjc.2023.104918. DOI: https://doi.org/10.1016/j.arabjc.2023.104918
[18] J. Tian et al., “Geographical origin verification of Crocus sativus L. grown using ‘two-segment’ cultivation,” Ind. Crops Prod., vol. 215, p. 118611, September 2024, https://doi.org/10.1016/j.indcrop.2024.118611. DOI: https://doi.org/10.1016/j.indcrop.2024.118611
[19] B. M. Razavizadeh and S. M. Ziaratnia, “Ultrasound treatment on saffron (Crocus sativus L.) corm: Impact on textural, morphological, and microbial properties and stigma-derived metabolite compositions,” Heliyon, vol. 10, no. 22, pp. 1–12, November 2024 https://doi.org/10.1016/j.heliyon.2024.e40548. DOI: https://doi.org/10.1016/j.heliyon.2024.e40548
[20] M. Anguera-Tejedor et al., “Exploring the therapeutic potential of bioactive compounds from selected plant extracts of Mediterranean diet constituents for cardiovascular diseases: A review of mechanisms of action, clinical evidence, and adverse effects”, Food Biosci., vol. 62, p. 105487, December 2024., https://doi.org/10.1016/j.bio.2024.105487. DOI: https://doi.org/10.1016/j.fbio.2024.105487
[21] J. Tan et al., “Extracts from petal of the Crocus sativus (saffron) possesses detoxification effects on acetaminophen induced liver injury by inhibiting hepatocyte apoptosis via regulating Nrf2/HO-1 signaling,” Fitoterapia, vol. 182, p. 106452, April 2025, https://doi.org/10.1016/j.fitote.2025.106452. DOI: https://doi.org/10.1016/j.fitote.2025.106452
[22] S. Liu, H. Guo, Z. Kong, X. Han, Y. Gao, and Y. Zhang, “Performance improvement and application of copper-based nanomaterials in membrane technology for water treatment : A review,” J. Environ. Manage., vol. 370, no. 10, p. 122755, November 2024, https://doi.org/10.1016/j.jenvman.2024.122755. DOI: https://doi.org/10.1016/j.jenvman.2024.122755
[23] A. García et al., “Copper-modified polymeric membranes for water treatment: A comprehensive review,” Membranes (Basel)., vol. 11, no. 2, January 2021, https://doi.org/10.3390/membranes11020093. DOI: https://doi.org/10.3390/membranes11020093
[24] M. Rabbani et al., “Nanocomposite membranes for water separation and purification : Fabrication , modification , and applications,” vol. 213, no. 9, pp. 465–499, April 2019, https://doi.org/10.1016/j.seppur.2018.12.050. DOI: https://doi.org/10.1016/j.seppur.2018.12.050
[25] A. Hosseinzadeh, J. L. Zhou, A. H. Navidpour, and A. Altaee, “Progress in osmotic membrane bioreactors research: Contaminant removal, microbial community and bioenergy production in wastewater,” Bioresour. Technol., vol. 330, p. 124998, June 2021, https://doi.org/10.1016/j.biortech.2021.124998. DOI: https://doi.org/10.1016/j.biortech.2021.124998
[26] F. Saffarimiandoab, B. Yavuzturk, and R. Sengur, “A review on membrane fouling : membrane modification,” vol. 216, pp. 47–70, March 2021, https://doi.org/10.5004/dwt.2021.26815. DOI: https://doi.org/10.5004/dwt.2021.26815
[27] A. Kim, J. Hak, and R. Patel, “Modification strategies of membranes with enhanced anti-biofouling properties for wastewater treatment : A review,” Bioresour. Technol., vol. 345, no. 10, p. 126501, February 2022, https://doi.org/10.1016/j.biortech.2021.126501. DOI: https://doi.org/10.1016/j.biortech.2021.126501
[28] N. M. Chauke, T. S. Munonde, and N. Mketo, “A critical review of the anti-biofouling properties of biogenic-based silver nanoparticles (AgNPs) embedded on polymer membranes for wastewater treatment,” J. Ind. Eng. Chem., to be published, https://doi.org/10.1016/j.jiec.2025.02.012. DOI: https://doi.org/10.1016/j.jiec.2025.02.012
[29] N. Wang et al., “Application of nanomaterials in antifouling : A review,” Nano Mater. Sci., no. 6, pp 672-700, December 2024, doi: 10.1016/j.nanoms.2024.01.009. DOI: https://doi.org/10.1016/j.nanoms.2024.01.009
[30] A. H. Sadek et al., “Polysulfone-based mixed matrix membranes loaded with a multifunctional hierarchical porous Ag-Cu dendrites@SiO2 core-shell nanostructure for wastewater treatment,” Process Saf. Environ. Prot., vol. 175, pp. 677–691, July 2023, https://doi.org/10.1016/j.psep.2023.05.085. DOI: https://doi.org/10.1016/j.psep.2023.05.085
[31] M. M. Armendáriz-Ontiveros, A. García-García, A. Mai-Prochnow, and G. A. Fimbres Weihs, “Optimal loading of iron nanoparticles on reverse osmosis membrane surface to reduce biofouling,” Desalination, vol. 540, p. 115997, October 2022, https://doi.org/10.1016/j.desal.2022.115997. DOI: https://doi.org/10.1016/j.desal.2022.115997
[32] L. Pang et al., “Antibiofouling thin-film nanocomposite membranes for sustainable water purification,” Adv. Sustain. Syst., vol. 5, no. 6, p. 2000279, June 2021, https://doi.org/10.1002/adsu.202000279. DOI: https://doi.org/10.1002/adsu.202000279
[33] M. Armendariz Ontiveros et al., “Anti-biofouling and desalination properties of thin film composite reverse osmosis membranes modified with copper and iron nanoparticles,” Materials, vol. 12, no. 13. June 2019, https://doi.org/10.3390/ma12132081. DOI: https://doi.org/10.3390/ma12132081
[34] Z. J. Parkerson, T. Le, P. Das, S. N. Mahmoodi, and M. R. Esfahani, “Cu-MOF-polydopamine-incorporated functionalized nanofiltration membranes for water treatment: effect of surficial adhesive modification techniques,” ACS ES&T Water, vol. 1, no. 2, pp. 430–439, February 2021, https://doi.org/10.1021/acsestwater.0c00173. DOI: https://doi.org/10.1021/acsestwater.0c00173
[35] K. Szymański et al., “Novel polyethersulfone ultrafiltration membranes modified with Cu/titanate nanotubes,” J. Water Process Eng., vol. 33, p. 101098, February 2020, https://doi.org/10.1016/j.jwpe.2019.101098. DOI: https://doi.org/10.1016/j.jwpe.2019.101098
[36] A. El-Gendi, F. A. Samhan, N. Ismail, and L. A. N. El-Dein, “Synergistic role of Ag nanoparticles and Cu nanorods dispersed on graphene on membrane desalination and biofouling,” J. Ind. Eng. Chem., vol. 65, pp. 127–136, September 2018, https://doi.org/10.1016/j.jiec.2018.04.021. DOI: https://doi.org/10.1016/j.jiec.2018.04.021
[37] M. T. Margarito et al., “Fabrication of polythiourea-copper complex composite membrane and its anti-fouling property,” in IOP Conf. Ser.: Mater. Sci. Eng., vol. 778, p. 012178, May 2020, https://doi.org/10.1088/1757-899X/778/1/012178. DOI: https://doi.org/10.1088/1757-899X/778/1/012178
[38] C. Liu et al., “Electroless deposition of copper nanoparticles integrates polydopamine coating on reverse osmosis membranes for efficient biofouling mitigation,” Water Res., vol. 217, p. 118375, June 2022, https://doi.org/10.1016/j.watres.2022.118375. DOI: https://doi.org/10.1016/j.watres.2022.118375
[39] B. Rodríguez, D. Oztürk, M. Rosales, M. Flores, and A. García, “Antibiofouling thin-film composite membranes (TFC) by in situ formation of Cu-(m-phenylenediamine) oligomer complex,” J. Mater. Sci., vol. 53, no. 9, pp. 6325–6338, January 2018, https://doi.org/10.1007/s10853-018-2039-4. DOI: https://doi.org/10.1007/s10853-018-2039-4
[40] R. Quezada, Y. Quintero, J. C. Salgado, H. Estay, and A. García, “Understanding the phenomenon of copper ions release from copper-modified TFC membranes: A mathematical and experimental methodology using shrinking core model,” Nanomaterials, vol. 10, no. 6, pp. 1–18, June 2020, https://doi.org/10.3390/nano10061130. DOI: https://doi.org/10.3390/nano10061130
[41] N. Misdan et al., “CuBTC metal organic framework incorporation for enhancing separation and antifouling properties of nanofiltration membrane,” Chem. Eng. Res. Des., vol. 148, pp. 227–239, August 2019, https://doi.org/10.1016/j.cherd.2019.06.004. DOI: https://doi.org/10.1016/j.cherd.2019.06.004
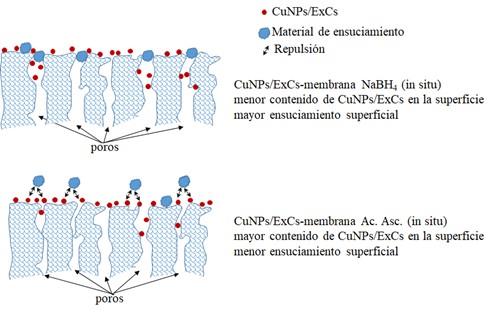
Published
How to Cite
Issue
Section
Categories
License
Copyright (c) 2025 Alfonso David Ibarra-Aguilar, Lucía Z. Flores-López, Heriberto Espinoza-Gómez, Jonatán Joel Aguirre-Camacho

This work is licensed under a Creative Commons Attribution 4.0 International License.
The authors who publish in this journal accept the following conditions:
The authors retain the copyright and assign to the journal the right of the first publication, with the work registered with the Creative Commons Attribution license 4.0, which allows third parties to use what is published as long as they mention the authorship of the work and the first publication in this magazine.
Authors may make other independent and additional contractual agreements for the non-exclusive distribution of the version of the article published in this journal (eg, include it in an institutional repository or publish it in a book) as long as they clearly indicate that the work it was first published in this magazine.
Authors are allowed and encouraged to share their work online (for example: in institutional repositories or personal web pages) before and during the manuscript submission process, as it can lead to productive exchanges, greater and more quick citation of published work (see The Effect of Open Access).